What are neutrinos and why are they worth the Nobel Prize in Physics?
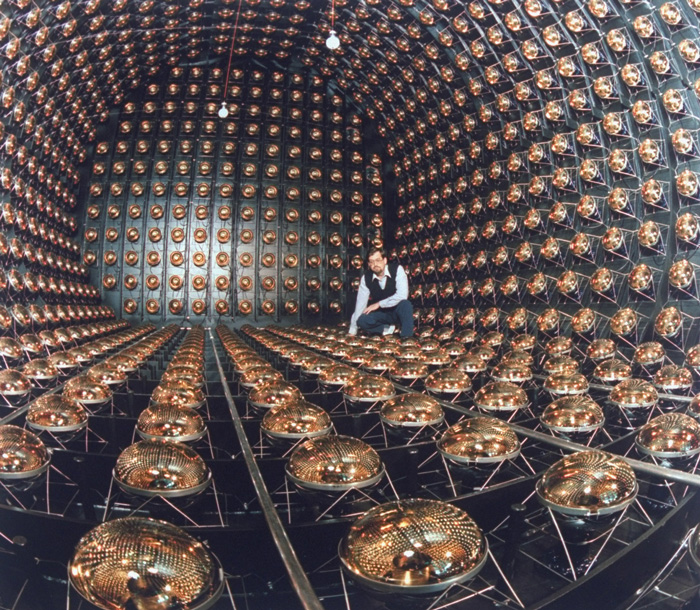
The Nobel Prize in Physics for this year has been jointly awarded to Takaaki Kajita and Arthur B McDonald, for proving that neutrinos - the second most common particles in the universe after photons - have mass and for explaining how these particles switch between different 'flavours'.
Kajita, 56, is the director of the Institute for Cosmic Ray Research and professor at the University of Tokyo. McDonald, 72, is a professor emeritus at Queen's University in Kingston, Canada.
It's an incredibly important discovery because it actually addresses an issue with the Standard Model of physics - a model recognised as broken years ago by scientists.
That's because by proving that neutrinos can change identities, or 'flavours' in scientific terms, it means they have mass.
The Standard Model had predicted they were massless, and it has no mechanism to provide them mass.
By uncovering the 'chameleon-like' nature of neutrinos, the laureates have solved a long-standing puzzle in particle physics that could alter our grasp of the cosmos, the Royal Swedish Academy of Sciences has said.
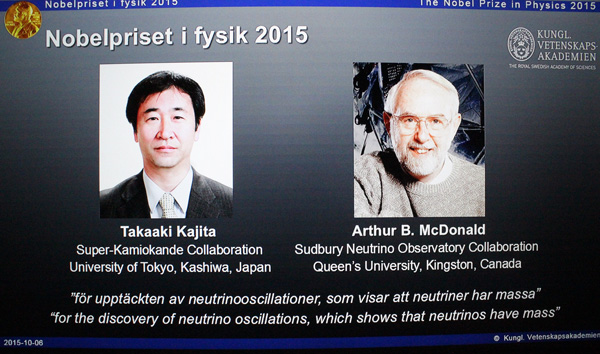
Photo: Atila Altuntas/Anadolu Agency/Getty Images
The Nobel Prize committee's statement reads: "The discovery has changed our understanding of the innermost workings of matter and can prove crucial to our view of the universe."
Following the announcement, the committee published a brief phone interview with Kajita.This is the point from where on most people's eyes will begin to glaze over. Neutrinos? What does that mean? Are they even remotely important?
The elusive neutrino
We already know the foundation: everyday matter is made up of combinations of 'elementary' particles called protons, neutrons and electrons. When they come together in different ways, they create any of the substances we see around us - from air to plastics to metal.
But there are many other particles that exist. They're unstable and exist only briefly before disappearing in a flash of energy. Of these, Neutrinos are possibly the most ghostlike.
Neutrinos are 'ubiquitous subatomic particles with almost no mass'. They're very difficult to study because they rarely interact with anything else. These subatomic particles are created from decayed radioactive elements.
Why are studying neutrinos essential?
According to Francis Halzen of the IceCube project, an Antarctica-based neutrino observatory, "Neutrinos are the ideal way to study the violent universe."
Basically, they are clues to the origins of cosmic rays - something that is still a mystery to scientists.
There's a huge chance that neutrinos are responsible for the very shape of the universe. When we look at other galaxies, we can see they have been pulled around by the gravity of some invisible clusters in space, which we call dark matter because it cannot be observed.
Could these be clusters of neutrinos?
The fact that neutrinos are so elusive also has some advantages.
Astronomers normally use telescopes to look at light. But light can be blocked by dust clouds or dense radiation fields in space between stars.
Neutrinos are subatomic particles that are created from decayed radioactive elements
But neutrinos can actually pass straight through all of this cosmic interference - giving us valuable information about what might be happening on the other side of the galaxy in the deep dark of space.
Theoretically, according to scientists, if the nuclear furnace that powers the sun switches off, it would be thousands of years before the sun would cool down enough for us to even take note of the fading light.
But because the neutrinos coming from the centre of the sun would stop immediately, scientists monitoring neutrinos would notice an event like that within minutes.
Where do neutrinos come from?
There are many sources in the universe - both in space and on Earth.
They are produced in the sun and other stars and are a byproduct of nuclear fusion, which involves the merging of two protons (hydrogen atoms) to form a deuteron, releasing a positron (anti-electron) and an electron neutrino at the same time.
Scientists believe that tonnes of neutrinos were created during the Big Bang.
In terms of man-made sources, nuclear reactors produce electron antineutrinos via beta decay.
Particle accelerators such as the Large Hadron Collider produce neutrinos of different flavours by firing protons into graphite or other targets.
The discovery
The first hint that these particles existed came in 1930 when a physicist - Wolfgang Pauli - noticed that in certain nuclear processes, some particles would just jump off in random directions - as if they'd been pushed.
He guessed that this was because there was another unseen particle - the neutrino - involved that was giving them a kick in the opposite direction. But at the time, there was no real method to prove the theory.
Fresh discoveries
Then in the 1990s, by when neutrinos could be detected, several physicists were faced with a conundrum that the couldn't crack: all their Earth-based detectors were picking out far fewer neutrinos than theoretical models predicted by scientists after Pauli.
These were calculations based on how many such particles should be produced by distant nuclear reactions, from our own sun to far-flung supernovas.
Neutrino detectors are generally huge volumes of fluid buried deep underground to avoid interference. When such a vast space is littered with light detectors, neutrinos can be glimpsed because of the tiny flashes of light that occur when they bump into an atom.
Such facilities include the Super-Kamiokande detector beneath Japan's Mount Kamioka, where Kajita still works, and the Sudbury Neutrino Observatory in Ontario, Canada, run by McDonald. Both are housed in disused mines.
In 1998, Kajita's team reported that the neutrinos they had caught, bouncing out of collisions in the Earth's atmosphere, had switched identity: were a different 'flavour' from what those collisions must have released.
This led them to wonder about what had made them change.
Then in 2001, a group led by McDonald announced that the neutrinos they were detecting in Ontario, which started out in the sun, had also 'flipped' from their expected identity.
This discovery of the particle's wobbly identity has crucial implications: it explains why neutrino detections had not matched the predicted quantities and changes calculations about the very nature of the universe, including its eternal expansion.
Still not 100% clear about neutrinos? Well, watch this 2010 lecture on the subject from the new Nobel laureate Arthur McDonald himself.
Breaking the neutrino down:
- It's the second most abundant particle in the Universe, after photons of light
- Means 'small, neutral one' in Italian
- Uncharged, and created in nuclear reactions and some radioactive decay chains
- Shown to have a tiny mass, but hardly interacts with other particles of matter
- Comes in three flavours, or types, referred to as muon, tau and electron
- These flavours are able to oscillate - flip from one type to another - during flight